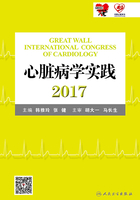
ApoB 与动脉粥样硬化的理论与证据
人类的载脂蛋白B(apolipoprotein B,ApoB)基因位于染色体2p24.1,基因全长43kb,由29个外显子和28个内含子组成。mRNA全长14 121个核苷酸,翻译原始ApoB100含有4563个氨基酸,是目前发现的较大的蛋白质之一。ApoB100蛋白质的分子量为512 937。但由于ApoB100有19个可能的N-糖苷化位点,故ApoB100是一个糖蛋白,含有10%左右的糖类。因此,整个ApoB100糖蛋白的分子量为540 000~550 000[1]。ApoB主要有两种形式存在:ApoB100和 ApoB48,还有少量的 ApoB90、ApoB80、ApoB72、ApoB53 等[2]。人体 ApoB100 表达于肝脏,存在于极低密度脂蛋白(very low density lipoproteins,VLDL)、中间密度脂蛋白(intermediate density lipoproteins,ILDL)和低密度脂蛋白(low density lipoproteins,LDL)上,而ApoB48在小肠表达,存在于乳糜微粒(chylomicrons,CM)中。ApoB作为结构性的载脂蛋白,也兼顾与受体结合的作用。但其与LDL受体(LDLR)亲和力较低,这也决定了LDL在血液中的保留时间较长。由于计算方法的不同,研究人员对ApoB100的二级结构说法不一,但也基本确定由α-螺旋、β-折叠、无规结构和β-转角等结构组成。ApoB100呈带状环绕着LDL,具有长而柔性的结构,其横截面约为2.0nm×5.4nm,长度约58.5nm[3]。研究人员将LDL分为外表层、界面层和内核,外表层主要由磷脂的亲水头部组成;界面层则由贯穿内核和表面层的脂类组成;内核则多为胆固醇酯,并不直接与表面单分子层接触。ApoB100在三个层次中均有分布[4](图1,见彩图1)。

ApoB存在于除高密度脂蛋白(HDL)之外的其他所有脂蛋白上,包括 VLDL、ILDL、LDL、脂蛋白(a)[Lp(a)]以及 CM[6](表1)。其中,CM在小肠中合成,VLDL由肝脏分泌,VLDL又分为VLDL1(较大,富含甘油三酯)和VLDL2(较小,含少量甘油三酯)。有文献报道,VLDL1的分泌增加血浆LDL水平,并且在胰岛素抵抗和Ⅱ型糖尿病患者中分泌增多[5]。VLDL由脂质核心(包括甘油三酯和胆固醇脂)以及外层的磷脂与游离胆固醇包裹。

在肝细胞中,由内质网核糖体合成的ApoB蛋白,进入内质网腔内并与微粒体甘油三酯转运蛋白(microsomal triglyceride transfer protein,MTP)结合形成类似口袋的结构,MTP促进甘油三酯与ApoB结合,并且将ApoB100的第3265到4082个氨基酸脂化,有利于形成VLDL前体(pre-VLDL)结构,pre-VLDL脂肪含量比较低,颗粒较小,密度较高,是不成熟的VLDL 颗粒。在这期间如果ApoB100的折叠发生错误,则会被chaperones binding protein(BiP)和protein disulfide isomerase(PDI)识别并保留在内质网中,然后进入蛋白质降解途径。随着pre-VLDL结合的脂质越来越多,形成较大的VLDL2,被分选到内质网出口(exit sites),在 SAR1(一个 GTPase)和外被体蛋白Ⅱ(coatamer proteinsⅡ,COP Ⅱ)的协助下,形成囊泡并且被转运到内质网与高尔基体的中间区域(ER-Golgi intermediate compartment,ERGIC)[7]。VLDL2囊泡可以运输到高尔基体的顺面膜囊(cis Golgi),但也可通过ARF1/COP Ⅰ转运回内质网。在高尔基体的VLDL2可以通过细胞内脂滴继续增加脂质,形成较大体积的VLDL1。经过高尔基体一系列的加工之后,VLDL2或VLDL1颗粒被分泌到细胞外[8](图2,见彩图2)。

VLDL的合成与分泌是非常复杂的过程,受到细胞中多种因子的调控,简单可分为蛋白质因素和脂肪因素[9]。ApoB作为结构蛋白以及转运脂肪所必需的 MTP是两种重要的调节因素,而在内质网确保ApoB正确折叠的蛋白如 Calreticulin、CaBP2、PDI、BiP、GRP94 等都是组装过程中重要的调节蛋白[10]。ApoE[11]、ApoC-Ⅲ[12]和ApoA-Ⅴ[13]也被报道可以调节ApoB的脂化过程。但是也有研究发现,在高尔基体中组装和分泌 VLDL 是只依赖于ApoB[7]。此外,脂肪合成、β氧化等调节肝脏脂肪含量的酶或转录因子也涉及调节VLDL 的合成与分泌。囊泡运输相关的蛋白如COPⅡ、COPⅠ、Sar1和ARF1等都会对VLDL在ER和Golgi之间的转运造成影响[14]。磷脂酶iPLA2β和PLD1、脂滴形成蛋白Cideb和ADRP能够调节细胞中脂肪的积累和 VLDL的合成与分泌[15]。胰岛素(insulin)信号通路也可以影响 VLDL 的合成与分泌[16]。除了蛋白因素,有效获得足够的脂肪是保证VLDL正常合成及充分脂化成熟的关键。所以,脂肪酸的合成以及甘油三酯的水平影响了VLDL合成与分泌的速度。VLDL在合成与分泌过程中受到如此多因素的调节,但VLDL并不是ApoB脂蛋白的唯一形式和主要功能脂蛋白,其在循环内的转化又是一个复杂的过程。
分泌的VLDL在进入血液后可由脂肪组织、肌肉组织的脂蛋白脂酶(lipoprotein lipase,LPL)水解其中的甘油三酯,HDL也转换胆固醇酯到VLDL,通过胆固醇酯转运蛋白(cholesteryl ester transfer protein,CETP)交换磷脂和甘油三酯。在LPL和CETP的作用下,使得越来越多的三酸甘油脂从VLDL被移走,这样使脂蛋白分子组成发生改变并成为IDL[17],大约半数的IDL将很快从血液循环中经过肝脏,释放出胆固醇用于制造新的VLDL和通过胆汁酸排出。没有被肝脏吸收的IDL继续留在血液循环中与肝脂酶(hepatic lipase,HL)结合进一步水解甘油三酯 ,经过一段时间后ApoE从IDL脱落,剩下的颗粒则转变为LDL(图3,见彩图3)。此时ApoB100占LDL中蛋白质含量的95%,ApoE和ApoC可能也存在于LDL上[18]。由于ApoB100与LDL受体的亲和力较低,LDL的寿命比IDL要长,它们在与肝脏和其他组织中的LDL受体结合前,通常在体内可以存在2.5天。ApoE的作用就是使得LDL尽快清除,具有积极的意义[1]。ApoE敲除鼠作为常见的动脉粥样硬化模型,也是基于ApoE清除脂蛋白的作用和机制。由于LDL存在异质性,其被研究者分为多个亚型且说法不一。Kraus和他的同事将LDL分为大而轻的LDL(large buoyant LDL,lbLDL)和小而密的 LDL(small dense LDL,sdLDL)[19]。而按照密度分类,可以分为 LDLⅠ~Ⅲ,即:LDLⅠ(large buoyant LDL)、LDL Ⅱ(intermediate density LDL)和 LDL Ⅲ(smaller dense LDL),密度分别为 1.025~1.034g/ml、1.034~1.044g/ml和1.044~1.060g/ml[20]。在CEPT的作用下,大颗粒VLDL中的甘油三酯与LDL和HDL中的胆固醇酯交换,LDL中的甘油三酯含量不断增加,胆固醇含量减少。形成大而轻的LDL,而当甘油三酯含量增加到一定水平后,更适合成为HL的底物,在HL的作用下形成sdLDL。sdLDL的形成与甘油三酯的含量密,高水平的甘油三酯可以促进VLDL和LDL间脂质的转换[21,22]。在全切相关基因组关联研究(GWAS)中发现,sortilin[23]、CETP、LPL、LIPC、GALNT2、MLXIPL、APOA1/A5 以及 PCSK7 的基因座 SNPs显示与sdLDL的水平和形成相关[24]。这说明sdLDL可能受这些基因的调节。

CM似乎和肝脏形成的ApoB脂蛋白没有直接联系,但CM从淋巴进入血液后也和其他脂蛋白进行脂质交换(见图3和彩图3),并且粥样斑块中也发现有CM存在的证据,说明其在动脉粥样硬化发生发展中起到了一定作用。ApoB48和ApoB100的mRNA是同一条序列,并不是剪接体。因为在人体小肠中存在一种RNA编辑酶APOBEC-1将原本编码ApoB100的mRNA在第6666个核苷酸由胞嘧啶变为尿嘧啶,导致在此处的CAA密码子被终止子 UAA替换。所以翻译在此处提前终止,就生成了只包含ApoB100 氨基端部分48%的ApoB48,含有2153个氨基酸。几乎所有的哺乳动物小肠都发生这种RNA编辑形式,但在小鼠、大鼠、马和狗的肝脏内也有发生,因此表现出较低的胆固醇水平,因而降低动脉粥样硬化的风险[25]。由于ApoB100的羧基端具有结合LDL受体的能力,而ApoB48不具有这段结合序列,也就无法被受体识别。但CM含有多个ApoE蛋白,其可以与LDLR结合。因此,CM相较只含有一个ApoB100的LDL颗粒更容易被受体识别并清除[26]。能够在上万核苷酸中对其中特定位点进行脱氨基作用,足以说明过程的精细。编辑位点附近是富含UA的高度保守序列[27],这需要多种蛋白因子共同作用、序列的精确性以及满足空间需求的二级结构[28]。其中,具有催化活性的亚基及其辅助因子是编辑复合体的核心酶。APOBEC-1是一类胞嘧啶核苷脱氨酶家族,是ApoB48的RNA编辑核心酶。ACF是一个65kDa大小的蛋白,在人类组织中分布广泛。有研究者敲除APOBEC-1基因的小鼠没有发现生命体征的损伤,而敲除ACF基因的小鼠却是致死的,说明ACF具有其他更重要的作用。研究报道,ACF蛋白有助于APOBEC-1向核内转运并识别ApoB mRNA的停泊序列最后由APOBEC-1完成脱氨基作用。当然这一精确单碱基修饰并不是只有这两种蛋白完成的。除了这两种关键蛋白,研究者确定了一系列有增强或抑制编辑活性的因子。其中,与APOBEC-1互作的包括hnRNP-C1、ABBP-2、GRY-RBP、CUGBP2、ABBP-1和hnRNP-A/B;KSRP与ACF相互作用[29]。人体肝脏主要合成ApoB100,如果肝脏也合成ApoB48结合在LDL上会使LDL在血浆中半衰期大大缩短,降低血脂水平。试图通过激活肝脏ApoB RNA编辑,成为了一个新的降脂手段。通过基因手段促进肝脏APOBEC-1表达后,血液ApoB100水平明显降低,但如何控制编辑比例是一项难题。小鼠过高表达APOBEC-1基因会导致6666个核苷酸后的多个位点受到编辑,且有患肝癌风险[30];而低水平表达APOBEC-1基因则有效避免了此类情况[31]。研究发现,雌激素、雄激素、甲状腺素、胰岛素、乙醇以及细胞质钙离子浓度等均被证明能够影响编辑的效率[32]。
虽然高LDL水平是目前致动脉粥样硬化的危险因素,但小肠CM也是动脉粥样硬化不容忽视的指标。越来越多证据发现,肠源性的CM及CM残粒在动脉粥样硬化的发生中也起着重要的作用[33];进一步研究还发现,ApoB48可能通过与动脉壁蛋白聚糖相互作用,介导包含ApoB48脂蛋白(主要是CM残粒)沉积于动脉壁[34]。
家族性高胆固醇血症(familial hypercholesterolemia,FH)被认为是动脉粥样硬化的独立危险因素,血浆内LDL-C高水平可能主要由三个基因的突变所导致(APOB、LDLR和PCSK9),但三个基因对于疾病对的贡献是不一致的(表2)。而且,ApoB基因突变造成的FH与LDLR基因突变的原理不尽相同[35]。

目前所发现的ApoB基因突变含有pre-VLDL脂化的区域(T71I、A591 V)[36,37],涉及VLDL向LDL转化的结构域(P2712L)[4],还有 LDLR 结合区域的突变(R3611Q、E4154K、N4311S)[38,39]。ApoB 的突变引起与LDLR结合位点的改变,包含ApoB的脂蛋白无法及时清除而导致FH。Arg-3500-Trp和Arg-3500-Gln位点的突变也会引起ApoB与LDLR的结合障碍,导致FH的发生[40]。研究发现Arg-3500-Trp/Gln会导致 ApoB 水平增加 44%(37.8mg/dl)并且 LDL-C 增加 80%(1.92mmol/L)[41,42] ,而 R3480P 减少 ApoB 水平 23%(19.9mg/dl)及降低 LDL-C 水平29%(0.93mmol/L)[40]。R3531C则没有改变 ApoB 和 LDL-C 水平[41] 。非编码区的 SNPs(rs531819、rs10199768、rs3791980、rs2678379)也证实与 ApoB 水平呈相关性[43]。
ApoB基因的多个SNPs在LDL水平相关的GWAS中被发现,rs562338、rs506585、rs515135、rs7575840等SNPs也在后续的实验中得以验证[44-47],rs693和rs1367117也证明与LDL水平呈相关性[48-50]。目前发现的ApoB的SNPs多为连锁平衡,只有P2712L和N4311S有高度的连锁不平衡,所以利用标签SNPs做基因分型研究可能不会全面分析其他SNPs的频率[43]。近期研究表明,SNP rs676210 GG相对于AA显著增加心肌梗死风险[odd ratio(OR)=1.93,95% 置信区间(CI):1.23~3.03]。单倍型分析显示 GTTGG(rs676210-rs2854725-rs11676704-rs3791980-rs2678379)单体型相对于ATTGA显著增加心肌梗死风险(OR=2.82,95%CI:1.49~5.33)。此外,ApoB基因rs676210和rs2678379与健康人群的血液ApoB水平呈相关性。这个实验表明,在中国汉族人群ApoB基因SNPs可能与心肌梗死和血液ApoB水平相关[51]。而研究发现,ApoB基因上 R463W 和 L343V 位点引起家族性低β脂蛋白血症(familial hypobetalipoproteinemia,FHBL)[52,53](图4,见彩图4)。ApoB基因突变或SNPs呈不利趋势的人群具有遗传特性,需要有针对的治疗手段,提早预防病变的发生。

引起LDL水平升高的因素极为复杂,包括ApoB的表达调控、VLDL装载与分泌、血液脂蛋白的相互转化、受体表达与调控、受体的再循环和降解、细胞脂质代谢水平、细胞外环境和激素等[54]。除了ApoB因素造成的血脂水平升高,研究人员在其他各个环节又展开了深入研究以降低血脂。有关LDL水平升高机制的综述较多,这里不再赘述。
虽然LDL的水平与动脉粥样硬化的发生、发展密切相关,但实际上初始的LDL并不会造成吞噬细胞的泡沫化(泡沫化细胞是动脉粥样硬化发展的重要阶段)(见图3和彩图3),原因是LDLR途径受细胞内固醇水平的反馈调节,当细胞内胆固醇水平升高时(巨噬细胞胆固醇主要从吞噬LDL后从溶酶体获得游离胆固醇)会抑制胆固醇调节元件结合蛋白(sterol-regulatory element binding proteins,SREBP)从内质网向高尔基体的转移,从而抑制了LDLR基因的转录,并且抑制LDLR从溶酶体向细胞膜的再循环。这样细胞膜上的LDLR水平降低,巨噬细胞就不会再内吞LDL[55](图5A,见彩图5A)。细胞内胆固醇水平得以控制,就不会发生泡沫化。然而,在血管内膜下的空间里,这个细胞对自身脂质水平的调控平衡,却被修饰的LDL所打破(图5B,见彩图5B)。在血液中LDL有丰富的抗氧化机制来对抗氧化作用,例如维生素 C、维生素 E[56]、谷胱甘肽过氧物酶[57]、对氧磷酶等[58],而当 LDL 进入内膜下,变为氧化过程为主导的环境,研究认为LDL氧化过程分为三类:第一阶段是抗氧化剂的消耗,包括维生素E和维生素C,这两类维生素可以从食物中补充,而内源性的维生素E需要谷胱甘肽和NADPH的参与;第二个阶段为进展阶段,多不饱和脂肪酸转变为共轭二烯、过氧化氢、烃醛复合物等;第三阶段烃醛复合物(醛或环氧化物)可以进一步分解生成丙二酰二醛(MDA)、4-羟壬烯醛(4-HNE)和己醛等。它们可进一步与ApoB100中的赖氨酸残基结合(LDLR结合位点含有丰富的赖氨酸),形成醛化LDL[59]。脂质的修饰可能也改变了ApoB的构象,造成错误的折叠,LDLR无法识别ApoB的结合区域,在氧化过程中ApoB的色氨酸残基不断暴露于水相,LDL呈现出负电荷(LDL-),这一亚类具有较强免疫反应性,促进淀粉样寡聚体抗体产生[60-62]。由于错误折叠,ApoB也可能从LDL上脱离下来,蛋白皱缩和聚集,不利于蛋白酶的分解。从圆二色谱分析,无脂质的ApoB的α-螺旋水平降低,无规则卷曲水平升高[63]。在许多研究中,无脂质的ApoB二级结构极为相似,而修饰LDL上的ApoB二级结构却因修饰程度和种类的不同而不同[62,64-66],这对ApoB的研究造成不小的困难。研究报道,无脂质的ApoB可以诱导J774巨噬细胞的凋亡。这种亲脂性的ApoB蛋白,极有可能是通过破坏细胞膜的结构,导致细胞死亡[63]。

研究表明,ApoB100的赖氨酸和精氨酸丰富的碱性氨基酸结构域位于3134~3209和3356~3489,这些碱性氨基酸可以和主动脉壁上的蛋白多糖结合。LPL作为ApoB与蛋白聚糖(pg)结合的桥梁分子[67,68]。在内膜下,巨噬细胞和平滑肌细胞都会分泌LPL[69,70]。ApoB100蛋白的B位点位于3359~3369个AA附近,可与蛋白多糖结合。1998年,Boren等鉴定了ApoB100的蛋白多糖结合位点发生突变,导致ApoB100滞留在动脉壁的比例减少,从而减少动脉粥样硬化的发生[71,72]。随后ApoB48也发现了蛋白多糖的结合位点[34],ApoB100 的 A 位点也相继发现(见图1 和彩图1),分泌的磷脂酶 A2(secreted phospholipase A2)修饰LDL之后形成sdLDL,A位点也得以暴露出来与蛋白多糖结合[73]。研究报道,sdLDL与蛋白多糖的亲和力是远远大于LDL的其他亚型[74]。研究报道,sdLDL与体内较高的甘油三酯相关,这种类型的LDL也普遍存在于肥胖、Ⅱ型糖尿病、代谢综合征患者体内。在绝经后的女性血液中,sdLDL的水平升高,且降低PON1的水平,使得sdLDL更容易被氧化[75]。
无论是蛋白多糖促进LDL的聚集使得自由基和氧化酶更容易氧化LDL,还是LDL本身氧化而促进LDL的聚集。最终导致的结果都是LDLR无法识别,取而代之的是被清道夫受体A-Ⅰ、B-Ⅰ、LRP、CD36以及toll样受体所识别。而这些受体均没有向LDLR那样对细胞内固醇水平的反馈调节,使得oxLDL不断的被巨噬细胞摄取形成泡沫化细胞,导致炎性分子释放和细胞凋亡,最终形成斑块内的坏死核心,同时也造成斑块的不稳定(见图5和彩图5)。oxLDL也可以促进淋巴细胞产生IgM抗体去识别氧化磷脂,说明oxLDL是具有免疫源性[76],抗原提呈细胞(巨噬细胞和树突状细胞为主)将修饰的LDL当做异物吞噬并将抗原信息提供给T淋巴细胞和B淋巴细胞。所以,在动脉粥样硬化中LDL的修饰不仅是天然免疫的结果,也激活了适应性免疫。
Lp(a)是LDL上的ApoB分子与Apo(a)以二硫键的形式结合形成的,Apo(a)的加入也可以认为是对LDL的一种修饰。Lp(a)的结构与LDL类似,Apo(a)是在肝脏合成的一个分子量非常大的蛋白,由若干环状结构组成[77]。Lp(a)已经成为动脉粥样硬化性心血管疾病的独立危险因素[78,79]。由于 Apo(a)的结构与纤维蛋白溶酶原类似,研究人员推测其与凝血瀑布反应相关[80]。而目前研究倾向于Lp(a)的脂质蛋白成分上,氧化磷脂的含量很高,这与动脉粥样硬化发展极为密切[81,82]。也许是氧化磷脂造成ApoB100结构的改变,使得与Apo(a)结合的二硫键更加暴露,又或许是Apo(a)结合到ApoB100促进了LDL的氧化。但无论是哪种因果关系,Lp(a)已经有大量证据证明是致动脉粥样硬化的。
LDL是一种异质性的颗粒,不但大小不一,而且脂类成分也随机体状态与进食相关。一些具有信号功能的脂类物质也存在于LDL颗粒上。神经酰胺是细胞的一种凋亡信号,也是鞘糖脂加工的物质基础,其分布于所有的脂蛋白上,而在斑块分离的LDL颗粒的神经酰胺含量是血液中LDL的50倍[83]。并且,LDL上神经酰胺的含量越高,LDL就越容易沉积在内膜下[84]。这说明LDL上的神经酰胺具有致动脉粥样硬化的作用。另一种鞘脂类物质,1-磷酸鞘氨醇(S1P)也被报道存在于LDL上[85],而且与神经酰胺具有相反作用[86]。Omega-3脂肪酸(十二碳五烯酸EPA和二十二碳六烯酸DHA)也被报道存在于LDL上。通过对人体LDL脂质组学分析,进食含有植物甾醇类和Omega-3脂肪酸添加的牛奶后,LDL的脂质成分发生改变,并且具有抗动脉粥样硬化作用[87]。上述也提到,甘油三酯和胆固醇在ApoB包含的脂蛋白中通过CEPT、LPL和HL等酶作用下进行相互传递,脂质种类和比例是动态的,是随饮食和机体状态密切相关的;也决定了LDL亚型的比例[21,22]。这些证据表明,脂质成分的不同也决定了ApoB包含的脂蛋白的抗/致动脉粥样硬化的性质。
餐后高血脂是动脉粥样硬化的独立危险因素[88],并且ApoB48水平也与动脉粥样硬化相关[89]。CM与CM残粒除了大小不同,脂质成分也有所区别。CM残粒游离胆固醇/甘油三酯的比例是CM的17倍[90]。而且CM残粒不同于LDL含有丰富的胆固醇酯,而是以游离胆固醇形式存在[91]。
CM由于体积较大不容易进入到内皮下,而CM残粒则满足体积的条件[92]。因为ApoB48缺少LDLR的结合位点,在血液和内皮下的CM残粒被ApoE识别。文献报道,LRP和HSPGs是识别并清除CM残粒的主要受体(见图5和彩图5),且不受细胞内胆固醇含量的调节[93]。CM残粒对于巨噬细胞和平滑肌细胞具有细胞毒性[94],可能的机制是CM残粒被受体内吞进入溶酶体后,由于自身含有较高的游离脂肪酸,破坏了溶酶体的膜结构,激活Bcl-2家族Bid蛋白的剪切从而导致凋亡[95,96]。也有报道显示,FC会激活caspase-3。CM致动脉粥样硬化的研究,相较于LDL少之又少。研究发现,ApoB48可能通过与动脉壁蛋白聚糖相互作用,介导包含ApoB48脂蛋白沉积于动脉壁[34]。
2016年发布的中国成人血脂异常防治指南指出总胆固醇、甘油三酯、LDL-C、HDL-C是主要的血脂指标,而ApoAⅠ、ApoB、Lp(a)的临床应用价值也日益受到关注[97]。LDL是被广泛用于预测动脉粥样硬化和缺血性心血管疾病的重要指标。相对于胆固醇水平,ApoB能够更好地预测心血管疾病的风险[98],因为ApoB存在于CM、VLDL、ILDL和LDL上且只有一分子。正常人群中,血清ApoB多在0.8~1.1g/L。除特殊说明外,临床常规测定的ApoB通常指的是ApoB100而不是ApoB48。血清ApoB主要反映LDL水平,与血清LDL-C水平呈明显正相关,两者的临床意义相似。在少数情况下,可出现高ApoB血症而LDL-C浓度正常的情况,说明血液中存在较多sdLDL(B型LDL)。当高甘油三酯血症时(VLDL高),sdLDL增高。与lbLDL(A型LDL)相比,sdLDL颗粒中ApoB含量较多而胆固醇较少,故可出现LDL-C虽然不高,但血清ApoB增高的所谓“高ApoB血症”,它反映B型LDL增多。所以,ApoB与LDL-C同时测定有利于临床判断[97]。
在一项用ApoB水平预测缺血性心脏病、心肌梗死和缺血性脑血管病风险的心脏研究中,在无性别差异的人群分析中发现较高ApoB人群相对于低ApoB人群的风险比分别为:缺血性心脏病1.8(95%CI:1.2~2.5)、心肌梗死 2.6(95%CI:1.4~4.7)和缺血性脑血管病 1.8(95%CI:1.3~2.3)。在男性分别为:缺血性心脏病1.9(95%CI:1.5~2.6)、心肌梗死 2.4(95%CI:1.5~3.6)、发生任何缺血性心血管病事件 1.6(95%CI:1.3~2.1)。ApoB 预测女性患病风险比分别为:缺血性脑血管病1.9(95%CI:1.2~2.9)和脑卒中 1.6(95%CI:1.0~2.8)[99]。
上海市一项6069例40岁以上人群健康调查,在校正性别、年龄以及传统心血管疾病因素后,ApoB(OR=1.23,95%CI:1.00~1.51)和 ApoB/ApoA-Ⅰ(OR=1.19,95%CI:1.04~1.36)与颈动脉内膜 -中膜厚度(CIMT)相关,能预测中年人群颈动脉狭窄[100]。
甘油三酯水平在200~499mg/dl的糖尿病人中,ApoB与非HDL-C指标的作用并不完全一致。甘油三酯高,导致LDL颗粒组成发生改变,LDL-C低估LDL-P的数量,进而不能准确地反映动脉粥硬化风险的程度。因此,甘油三酯水平(200~499mg/dl)需要用ApoB水平评估动脉粥样硬化风险[101]。
2013年FDA批准的27个新药中,米泊美生钠注射液(mipomersen)是Genzyme研发的一种合成的硫代磷酸寡核苷酸,被FDA批准用于治疗纯合子型家族性高胆固醇血症(homozygous familial hypercholesterolemia,FoFH)。作为反义核酸类药物,米泊美生通过与ApoB100蛋白mRNA的编码区互补配对,抑制ApoB100蛋白的翻译合成,降低FoFH患者的LDL-C、甘油三酯、non-HDL-C水平。在为期26周涉及56名FoFH的多国随机对照试验中,治疗组平均LDL-C、甘油三酯、ApoB、non-HDL-C、TG水平分别降低 25mg/dl、21mg/dl、27mg/dl、25mg/dl、18mg/dl,平均 HDL-C 水平增加 15mg/dl,而安慰剂组各项指标变化均在5mg/dl以内。值得注意的是,该药说明书中有一黑框警告,须警惕肝毒性[102,103]。
Lomitapide是MTP的一个抑制剂,可以降低LDL-C、甘油三酯和ApoB水平,但对降低Lp(a)没有效果[104]。3.4g/d EPA+DHA omega-3脂肪酸降低甘油三酯,降低ApoB水平4%。最新的ACCE指南——血脂异常管理与动脉粥样硬化预防总结了一些降脂类药物(表3)。

在过去20年里,动脉粥样硬化的炎症学说越来越受到研究者的认同。修饰LDL的高滴度的IgG和IgM 抗体也在临床研究中发现[105,106]。三种 ApoB 的肽段被应用于研究中,P45(ApoB100661~680)[107]、P143(ApoB100 2131~2150)和 P210(ApoB100 3136~3155)[108]。 免 疫 P45 和 P210 后,发现心脏 疾 病的患者的特异性IgG滴度明显低于健康人群[109,110],表明ApoB100的IgG是具有抗动脉粥样硬化性质的。在一些基于转基因小鼠的免疫研究发现,给小鼠免疫P45、P143或P210会不同程度降低斑块数量(45%~60%),同时IFN-γ对小鼠的免疫起到了促进作用[107,108,111]。用P210或修饰的P210刺激树突状细胞,可以激活CD4+CD25+Foxp3+Treg细胞,抑制动脉粥样硬化的发展[112]。IL-4和其他抗动脉粥样硬化的细胞因子也在ApoB免疫的小鼠中检测到[113]。最直接的证据是ApoB100 3501~3516[SQEYSGSVANEANVY]和ApoB100 978~993[TGAYSNASSTESASY]肽段和MHCⅡ分子是高度亲和的,说明抗原提呈细胞可以识别ApoB肽段以激活适应性免疫[114]。虽然众多研究证明,疫苗干预ApoB水平是一个抑制动脉粥样硬化的有效方法,但仍需要深入了解ApoB免疫原性的原理,以更早服务于临床[115]。
microRNA的研究进展主要集中在LDLR、PCSK9、MTP等涉及调节LDL水平的重要分子。最近的研究报道,miR-548p减少ApoB的分泌,通过结合其mRNA并抑制ApoB的表达[116]。过表达miR-122(靶点为 MTP)[117]、miR-34a(靶点为 HNF4α和 ApoB)[118]和 miR-30c(靶点为 MTP)[119]也可有效抑制包含ApoB脂蛋白的水平。通过Miranda和miRTarBase 数据库的预测,也得到了一些潜在的可以调节ApoB表达的miRNA,但仍需要研究鉴定,包括hsa-miR-885-5p、hsa-miR-7151-5p、hsa-miR-6869-5p、hsa-miR-502-5p、hsa-miR-1911-5p、hsa-miR-499b-5p、hsa-miR-500b-3p、hsa-miR-6862-3p、hsa-miR-6784-3p和hsa-miR-624-5p等[120]。Mipomersen药物同样基于mRNA抑制的原理而设计的。虽然miRNAs对于ApoB基因或相关蛋白的作用是直接的,但miRNAs具有多靶点的性质,如何评估一个miRNA对于LDL水平的影响还要分析其对多个基因抑制后的综合效果。
针对ApoB以及包含ApoB脂蛋白水平的药物或者基因干预,一个核心理念就是认为高水平的ApoB和它的脂蛋白是对人体不利的。以单纯降低ApoB水平的方法确实对预防动脉粥样硬化的发生起到了显著的效果。然而,这样的方法并不能完全适用于每一个个体。复杂的脂质运输本应该是外周组织获取脂类物质的正规途径。ApoB可能只是“一艘货船”,而“货物”的种类或者“港口”的需求也决定了ApoB的命运。在异质性的脂蛋白颗粒中,研究人员已经发现信号类脂质或者有生物活性的脂类物质往往决定了脂蛋白的作用。对脂蛋白评估好与坏的同时,更加关注脂蛋白成分将是未来临床指标的方向和难点。相信随着研究的不断深入、人为干预的全面化,很可能会去除脂蛋白的好与坏之分,还ApoB以清白。
(刘行 李广平)
1.卢玲,何炳林,袁直,等. 低密度脂蛋白结构研究进展. 离子交换与吸附,2001(5):363-368.
2.Stillemark-Billton P,Beck C,BorenJ,et al. Relation of the size and intracellular sorting of apoB to the formation of VLDL 1 and VLDL 2. J Lipid Res,2005,46(1):104-114.
3.van Antwerpen R,Chen GC,Pullinger CR,et al. Cryo-electron microscopy of low density lipoprotein and reconstituted discoidal high density lipoprotein:imaging of the apolipoprotein moiety. J Lipid Res,1997,38(4):659-669.
4.Hevonoja T,Pentikainen MO,Hyvonen MT,et al. Structure of low density lipoprotein(LDL) particles:basis for understanding molecular changes in modified LDL. Biochim Biophys Acta,2000,1488(3):189-210.
5.Taskinen MR. Diabetic dyslipidaemia:from basic research to clinical practice . Diabetologia,2003,46(6):733-749.
6.Mahley RW,Ji ZS. Remnant lipoprotein metabolism:key pathways involving cell-surface heparan sulfate proteoglycans and apolipoprotein E. J Lipid Res,1999,40(1):1-16.
7.Gusarova V,Seo J,Sullivan ML,et al. Golgi-associated maturation of very low density lipoproteins involves conformational changes in apolipoprotein B,but is not dependent on apolipoprotein E. J Biol Chem,2007,282(27):19453-19462.
8.Kartberg F,Elsner M,Froderberg L,et al. Commuting between Golgi cisternae--mind the GAP!. Biochim Biophys Acta,2005,1744(3):351-363.
9.Sundaram M,Yao Z. Recent progress in understanding protein and lipid factors affecting hepatic VLDL assembly and secretion. Nutr Metab(Lond),2010(7):35.
10.Olofsson SO,Stillemark-Billton P,Asp L. Intracellular assembly of VLDL:two major steps in separate cell compartments. Trends Cardiovasc Med,2000,10(8):338-345.
11.Huang Y,Liu XQ,Rall SC Jr,et al. Overexpression and accumulation of apolipoprotein E as a cause of hypertriglyceridemia. J Biol Chem,1998,273(41):26388-26393.
12.van Dijk KW,Rensen PC,Voshol PJ,et al. The role and mode of action of apolipoproteins CⅢ and AⅤ:synergistic actors in triglyceride metabolism ?. Curr Opin Lipidol,2004,15(3):239-246.
13.Schaap FG,Rensen PC,Voshol PJ,et al. ApoAV reduces plasma triglycerides by inhibiting very low density lipoprotein-triglyceride(VLDL-TG)production and stimulating lipoprotein lipase-mediated VLDL-TG hydrolysis. J Biol Chem,2004,279(27):27941-27947.
14.李烜赫. Cideb和ADRP调控肝脏VLDL脂化成熟及脂稳态的作用研究. 清华大学,2012.
15.Tran K,Sun F,Cui Z,et al. Attenuated secretion of very low density lipoproteins from McA-RH7777 cells treated with eicosapentaenoic acid is associated with impaired utilization of triacylglycerol synthesized via phospholipid remodeling. Biochim Biophys Acta,2006,1761(4):463-473.
16.Meshkani R,Adeli K. Hepatic insulin resistance,metabolic syndrome and cardiovascular disease. Clin Biochem,2009,42(13-14):1331-1346.
17.Shelness GS,Sellers JA. Very-low-density lipoprotein assembly and secretion. Curr Opin Lipidol,2001,12(2):151-157.
18.Sacks FM. The crucial roles of apolipoproteins E and C-Ⅲ in apoB lipoprotein metabolism in normolipidemia and hypertriglyceridemia. Curr Opin Lipidol,2015,26(1):56-63.
19.Krauss RM. Lipoprotein subfractions and cardiovascular disease risk. Curr Opin Lipidol,2010,21(4):305-311.
20.Griffin BA,Caslake MJ,Yip B,et al. Rapid isolation of low density lipoprotein(LDL) subfractions from plasma by density gradient ultracentrifugation. Atherosclerosis,1990,83(1):59-67.
21.Ivanova EA,Myasoedova VA,Melnichenko AA,et al. Small Dense Low-Density Lipoprotein as Biomarker for Atherosclerotic Diseases. Oxid Med Cell Longev,2017(2017):1273042.
22.Brousseau ME,Schaefer EJ,Wolfe ML,et al. Effects of an inhibitor of cholesteryl ester transfer protein on HDL cholesterol. N Engl J Med,2004,350(15):1505-1515.
23.Musunuru K,Strong A,Frank-Kamenetsky M,et al. From noncoding variant to phenotype via SORT1 at the 1p13 cholesterol locus. Nature,2010,466(7307):714-719.
24.Hoogeveen RC,Gaubatz JW,Sun W,et al. Small dense low-density lipoprotein-cholesterol concentrations predict risk for coronary heart disease:the Atherosclerosis Risk In Communities(ARIC) study. Arterioscler Thromb Vasc Biol,2014,34(5):1069-1077.
25.Greeve J,Altkemper I,Dieterich JH,et al. Apolipoprotein B mRNA editing in 12 different mammalian species:hepatic expression is reflected in low concentrations of apoB-containing plasma lipoproteins. J Lipid Res,1993,34(8):1367-1383.
26.Borensztajn J,Kotlar TJ. Liver uptake of chylomicron remnants with high and low apoprotein E:C ratios. Proc Natl Acad Sci U SA,1984,81(18):5863-5866.
27.张森,李辉 . 载脂蛋白 B 研究进展 . 国际遗传学杂志,2006(05):364-367.
28.Blanc V,Kennedy S,Davidson NO. A novel nuclear localization signal in the auxiliary domain of apobec-1 complementation factor regulates nucleocytoplasmic import and shuttling. J Biol Chem,2003,278(42):41198-41204.
29.刘树涛,李慧萍,齐庆远 . 载脂蛋白 B RNA 编辑机制概述 . 中国农学通报,2013(15):135-141.
30.Hersberger M,Patarroyo-White S,Qian X,et al. Regulatable liver expression of the rabbit apolipoprotein B mRNA-editing enzyme catalytic polypeptide 1(APOBEC-1) in mice lacking endogenous APOBEC-1 leads to aberrant hyperediting. Biochem J,2003,369(Pt 2):255-262.
31.Yang Y,Ballatori N,Smith HC. Apolipoprotein B mRNA editing and the reduction in synthesis and secretion of the atherogenic risk factor,apolipoprotein B100 can be effectively targeted through TAT-mediated protein transduction. Mol Pharmacol,2002,61(2):269-276.
32.Levy E,Sinnett D,Thibault L,et al. Insulin modulation of newly synthesized apolipoproteins B-100 and B-48 in human fetal intestine:gene expression and mRNA editing are not involved. FEBS Lett,1996,393(2-3):253-258.
33.Fujioka Y,Ishikawa Y. Remnant lipoproteins as strong key particles to atherogenesis. J Atheroscler Thromb,2009,16(3):145-154.
34.Flood C,Gustafsson M,Richardson PE,et al. Identification of the proteoglycan binding site in apolipoprotein B48. J Biol Chem,2002,277(35):32228-32233.
35.Youngblom E,Pariani M,Knowles JW. Familial Hypercholesterolemia.Seattle(WA):Gene Reviews(R),1993.
36.Mann CJ,Anderson TA,Read J,et al. The structure of vitellogenin provides a molecular model for the assembly and secretion of atherogenic lipoproteins. J Mol Biol,1999,285(1):391-408.
37.Bradbury P,Mann CJ,Kochl S,et al. A common binding site on the microsomal triglyceride transfer protein for apolipoprotein B and protein disulfide isomerase. J Biol Chem,1999,274(5):3159-3164.
38.Chatterton JE,Phillips ML,Curtiss LK,et al. Immunoelectron microscopy of low density lipoproteins yields a ribbon and bow model for the conformation of apolipoprotein B on the lipoprotein surface. J Lipid Res,1995,36(9):2027-2037.
39.Boren J,Lee I,Zhu W,et al. Identification of the low density lipoprotein receptor-binding site in apolipoprotein B100 and the modulation of its binding activity by the carboxyl terminus in familial defective apo-B100. J Clin Invest,1998,101(5):1084-1093.
40.Benn M,Nordestgaard BG,Jensen JS,et al. Mutation in apolipoprotein B associated with hypobetalipoproteinemia despite decreased binding to the low density lipoprotein receptor. J Biol Chem,2005,280(22):21052-21060.
41.Tybjaerg-Hansen A,Steffensen R,Meinertz H,et al. Association of mutations in the apolipoprotein B gene with hypercholesterolemia and the risk of ischemic heart disease. N Engl J Med,1998,338(22):1577-1584.
42.Tybjaerg-Hansen A,Jensen HK,Benn M,et al. Phenotype of heterozygotes for low-density lipoprotein receptor mutations identified in different background populations. Arterioscler,Thromb Vasc Biol,2005,25(1):211-215.
43.Benn M. Apolipoprotein B levels,APOB alleles,and risk of ischemic cardiovascular disease in the general population,a review. Atherosclerosis,2009,206(1):17-30.
44.Chasman DI,Pare G,Zee RY,et al. Genetic loci associated with plasma concentration of low-density lipoprotein cholesterol,high-density lipoprotein cholesterol,triglycerides,apolipoprotein A1,and Apolipoprotein B among 6382 white women in genome-wide analysis with replication.Circ Cardiovasc Genet,2008,1(1):21-30.
45.Wallace C,Newhouse SJ,Braund P,et al. Genome-wide association study identifies genes for biomarkers of cardiovascular disease:serum urate and dyslipidemia. Am J Human Genet,2008,82(1):139-149.
46.Aulchenko YS,Ripatti S,Lindqvist I,et al. Loci influencing lipid levels and coronary heart disease risk in 16 European population cohorts. Nat Genet,2009,41(1):47-55.
47.Chasman DI,Pare G,Mora S,et al. Forty-three loci associated with plasma lipoprotein size,concentration,and cholesterol content in genome-wide analysis. PLoS Genet,2009,5(11):e1000730.
48.Rodrigues AC,Sobrino B,Genvigir FD,et al. Genetic variants in genes related to lipid metabolism and atherosclerosis,dyslipidemia and atorvastatin response. Clin Chim Acta,2013,(417):8-11.
49.Park MH,Kim N,Lee JY,et al. Genetic loci associated with lipid concentrations and cardiovascular risk factors in the Korean population. J Med Genet,2011,48(1):10-15.
50.Kathiresan S,Melander O,Anevski D,et al. Polymorphisms associated with cholesterol and risk of cardiovascular events. N Engl J Med,2008,358(12):1240-1249.
51.Liu C,Yang J,Han W,et al. Polymorphisms in ApoB gene are associated with risk of myocardial infarction and serum ApoB levels in a Chinese population. Int J Clin Exp Med,2015,8(9):16571-16577.
52.Noto D,Cefalu AB,Cannizzaro A,et al. Familial hypobetalipoproteinemia due to apolipoprotein B R463W mutation causes intestinal fat accumulation and low postprandial lipemia. Atherosclerosis,2009,206(1):193-198.
53.Hooper AJ,Heeks L,Robertson K,et al. Lipoprotein Metabolism in APOB L343V Familial Hypobetalipoproteinemia. J Clin Endocrinol Metab,2015,100(11):E1484-E1490.
54.Linton MF,Yancey PG,Davies SS,et al. The Role of Lipids and Lipoproteins in Atherosclerosis.South Dartmouth(MA):Endotext,2000.
55.Goldstein JL,Debose-Boyd RA,Brown MS. Protein sensors for membrane sterols. Cell,2006,124(1):35-46.
56.Mols P,Hallemans R,Melot C,et al. Systemic and regional hemodynamic effects of isosorbide dinitrate in patients with liver cirrhosis and portal hypertension. J Hepatol,1989,8(3):316-324.
57.Hussein O,Rosenblat M,Refael G,et al. Dietary selenium increases cellular glutathione peroxidase activity and reduces the enhanced susceptibility to lipid peroxidation of plasma and low-density lipoprotein in kidney transplant recipients. Transplantation,1997,63(5):679-685.
58.Durrington PN,Mackness B,Mackness MI. Paraoxonase and atherosclerosis. Arterioscler Thromb Vasc Biol,2001,21(4):473-480.
59.倪敬年,时晶,田金洲. LDL的化学修饰及其致动脉粥样硬化作用. 中国老年学杂志,2006(11):1586-1588.
60.Stewart CR,Tseng AA,Mok YF,et al. Oxidation of low-density lipoproteins induces amyloid-like structures that are recognized by macrophages.Biochemistry,2005,44(25):9108-9116.
61.Asatryan L,Hamilton RT,Isas JM,et al. LDL phospholipid hydrolysis produces modified electronegative particles with an unfolded apoB-100 protein. J Lipid Res,2005,46(1):115-122.
62.Parasassi T,De Spirito M,Mei G,et al. Low density lipoprotein misfolding and amyloidogenesis. FASEB J,2008,22(7):2350-2356.
63.Morita SY,Deharu Y,Takata E,et al. Cytotoxicity of lipid-free apolipoprotein B. Biochim Biophys Acta,2008,1778(11):2594-2603.
64.Parasassi T,Bittolo-Bon G,Brunelli R,et al. Loss of apoB-100 secondary structure and conformation in hydroperoxide rich,electronegative LDL(-).Free Radic Biol Med,2001,31(1):82-89.
65.Brunelli R,Mei G,Krasnowska EK,et al. Estradiol enhances the resistance of LDL to oxidation by stabilizing apoB-100 conformation.Biochemistry,2000,39(45):13897-13903.
66.Jayaraman S,Gantz DL,Gursky O. Effects of oxidation on the structure and stability of human low-density lipoprotein. Biochemistry,2007,46(19):5790-5797.
67.Curcio CA,Johnson M,Huang JD,et al. Apolipoprotein B-containing lipoproteins in retinal aging and age-related macular degeneration. J Lipid Res,2010,51(3):451-467.
68.Oorni K,Pentikainen MO,Ala-Korpela M,et al. Aggregation,fusion,and vesicle formation of modified low density lipoprotein particles:molecular mechanisms and effects on matrix interactions. J Lipid Res,2000,41(11):1703-1714.
69.Yla-Herttuala S,Lipton BA,Rosenfeld ME,et al. Macrophages and smooth muscle cells express lipoprotein lipase in human and rabbit atherosclerotic lesions. Proc Natl Acad Sci U S A,1991,88(22):10143-10147.
70.O’Brien KD,Gordon D,Deeb S,et al. Lipoprotein lipase is synthesized by macrophage-derived foam cells in human coronary atherosclerotic plaques. J Clin Invest,1992,89(5):1544-1550.
71.Boren J,Olin K,Lee I,et al. Identification of the principal proteoglycan-binding site in LDL. A single-point mutation in apo-B100 severely affects proteoglycan interaction without affecting LDL receptor binding. J Clin Invest,1998,101(12):2658-2664.
72.Skalen K,Gustafsson M,Rydberg EK,et al. Subendothelial retention of atherogenic lipoproteins in early atherosclerosis. Nature,2002,417(6890):750-754.
73.Flood C,Gustafsson M,Pitas RE,et al. Molecular mechanism for changes in proteoglycan binding on compositional changes of the core and the surface of low-density lipoprotein-containing human apolipoprotein B100. Arterioscler Thromb Vasc Biol,2004,24(3):564-570.
74.Anber V,Griffin BA,Mcconnell M,et al. Influence of plasma lipid and LDL-subfraction profile on the interaction between low density lipoprotein with human arterial wall proteoglycans. Atherosclerosis,1996,124(2):261-271.
75.Mogarekar MR,Kulkarni SK. Small Dense Low Density Lipoprotein Cholesterol,Paraoxonase 1 and Lipid Profile in Postmenopausal Women:Quality or Quantity ? . Arch Med Res,2015,46(7):534-538.
76.Huang YH,Ronnelid J,Frostegard J. Oxidized LDL induces enhanced antibody formation and MHC class Ⅱ-dependent IFN-gamma production in lymphocytes from healthy individuals. Arterioscler Thromb Vasc Biol,1995,15(10):1577-1583.
77.Mcneal C. Lipoprotein(A). South Dartmouth(MA):Endotext,2000.
78.Emerging Risk Factors Collaboration,Erqou S,Kaptoge S,et al. Lipoprotein(a) concentration and the risk of coronary heart disease,stroke,and nonvascular mortality. JAMA,2009,302(4):412-423.
79.Boffa MB,Koschinsky ML. Lipoprotein(a):truly a direct prothrombotic factor in cardiovascular disease?. J Lipid Res,2016,57(5):745-757.
80.Angles-Cano E,Hervio L,Rouy D,et al. Effects of lipoprotein(a) on the binding of plasminogen to fibrin and its activation by fibrin-bound tissuetype plasminogen activator. Chem Phys Lipids,1994,67-68:369-380.
81.Capoulade R,Chan KL,Yeang C,et al. Oxidized Phospholipids,Lipoprotein(a),and Progression of Calcific Aortic Valve Stenosis. J Am Coll Cardiol,2015,66(11):1236-1246.
82.Witztum JL,Ginsberg HN. Lipoprotein(a):Coming of Age at Last. J Lipid Res,2016,57(3):336-339.
83.Schissel SL,Tweedie-Hardman J,Rapp JH,et al. Rabbit aorta and human atherosclerotic lesions hydrolyze the sphingomyelin of retained lowdensity lipoprotein. Proposed role for arterial-wall sphingomyelinase in subendothelial retention and aggregation of atherogenic lipoproteins. J Clin Invest,1996,98(6):1455-1464.
84.Li W,Yang X,Xing S,et al. Endogenous ceramide contributes to the transcytosis of oxLDL across endothelial cells and promotes its subendothelial retention in vascular wall. Oxid Med Cell Longev,2014,2014:823071.
85.Murata N,Sato K,Kon J,et al. Interaction of sphingosine 1-phosphate with plasma components,including lipoproteins,regulates the lipid receptormediated actions. Biochem J,2000,352(Pt 3):809-815.
86.Liu X,Zhang QH,Yi GH. Regulation of metabolism and transport of sphingosine-1-phosphate in mammalian cells. Mol Cell Biochem,2012,363(1-2):21-33.
87.Padro T,Vilahur G,Sanchez-Hernandez J,et al. Lipidomic changes of LDL in overweight and moderately hypercholesterolemic subjects taking phytosterol- and omega-3-supplemented milk. J Lipid Res,2015,56(5):1043-1056.
88.Goldberg IJ,Kako Y,Lutz EP. Responses to eating:lipoproteins,lipolytic products and atherosclerosis. Curr Opin Lipidol,2000,11(3):235-241.
89.Masuda D,Sugimoto T,Tsujii K,et al. Correlation of fasting serum apolipoprotein B-48 with coronary artery disease prevalence. Eur J Clin Invest,2012,42(9):992-999.
90.Kawasaki S,Taniguchi T,Fujioka Y,et al. Chylomicron remnant induces apoptosis in vascular endothelial cells. Ann N Y Acad Sci,2000,(902):336-341.
91.Fielding CJ. Lipoprotein receptors,plasma cholesterol metabolism,and the regulation of cellular free cholesterol concentration. FASEB J,1992,6(13):3162-3168.
92.Proctor SD,Vine DF,Mamo JC. Arterial retention of apolipoprotein B(48)- and B(100)-containing lipoproteins in atherogenesis. Curr Opin Lipidol,2002,13(5):461-470.
93.Hsu HY,Hajjar DP,Khan KM,et al. Ligand binding to macrophage scavenger receptor-A induces urokinase-type plasminogen activator expression by a protein kinase-dependent signaling pathway. J Biol Chem,1998,273(2):1240-1246.
94.Yu KC,Mamo JC. Chylomicron-remnant-induced foam cell formation and cytotoxicity:a possible mechanism of cell death in atherosclerosis. Clin Sci,2000,98(2):183-192.
95.Guicciardi ME,Deussing J,Miyoshi H,et al. Cathepsin B contributes to TNF-alpha-mediated hepatocyte apoptosis by promoting mitochondrial release of cytochrome c. J Clin Invest,2000,106(9):1127-1137.
96.Stoka V,Turk B,Schendel SL,et al. Lysosomal protease pathways to apoptosis. Cleavage of bid,not pro-caspases,is the most likely route. J Biol Chem,2001,276(5):3149-3157.
97.诸骏仁,高润霖,赵水平,等 . 中国成人血脂异常防治指南(2016 年修订版). 中国循环杂志,2016(10):937-953.
98.Mcqueen MJ,Hawken S,Wang X,et al. Lipids,lipoproteins,and apolipoproteins as risk markers of myocardial infarction in 52 countries(the INTERHEART study):a case-control study. Lancet,2008,372(9634):224-233.
99.Benn M,Nordestgaard BG,Jensen GB,et al. Improving prediction of ischemic cardiovascular disease in the general population using apolipoprotein B:the Copenhagen City Heart Study. Arterioscler Thromb Vasc Biol,2007,27(3):661-670.
100.Huang F,Yang Z,Xu B,et al. Both serum apolipoprotein B and the apolipoprotein B/apolipoprotein A-I ratio are associated with carotid intimamedia thickness. PloS One,2013,8(1):e54628.
101.Ganda OP,Jumes CG,Abrahamson MJ,et al. Quantification of concordance and discordance between apolipoprotein-B and the currently recommended non-HDL-cholesterol goals for cardiovascular risk assessment in patients with diabetes and hypertriglyceridemia. Diabetes Res Clin Pract,2012,97(1):51-56.
102.Stein EA,Dufour R,Gagne C,et al. Apolipoprotein B synthesis inhibition with mipomersen in heterozygous familial hypercholesterolemia:results of a randomized,double-blind,placebo-controlled trial to assess efficacy and safety as add-on therapy in patients with coronary artery disease.Circulation,2012,126(19):2283-2292.
103.Raal FJ,Santos RD,Blom DJ,et al. Mipomersen,an apolipoprotein B synthesis inhibitor,for lowering of LDL cholesterol concentrations in patients with homozygous familial hyper- cholesterolaemia:a randomised,double-blind,placebo-controlled trial. Lancet,2010,375(9719):998-1006.
104.Cuchel M,Meagher EA,Du Toit Theron H,et al. Efficacy and safety of a microsomal triglyceride transfer protein inhibitor in patients with homozygous familial hypercholesterolaemia:a single-arm,open-label,phase 3 study. Lancet,2013,381(9860):40-46.
105.Gounopoulos P,Merki E,Hansen LF,et al. Antibodies to oxidized low density lipoprotein:epidemiological studies and potential clinical applications in cardiovascular disease. Minerva Cardioangiol,2007,55(6):821-837.
106.Ravandi A,Boekholdt SM,Mallat Z,et al. Relationship of IgG and IgM autoantibodies and immune complexes to oxidized LDL with markers of oxidation and inflammation and cardiovascular events:results from the EPIC-Norfolk Study. J Lipid Res,2011,52(10):1829-1836.
107.Fredrikson GN,Andersson L,Soderberg I,et al. Atheroprotective immunization with MDA-modified apo B-100 peptide sequences is associated with activation of Th2 specific antibody expression. Autoimmunity,2005,38(2):171-179.
108.Fredrikson GN,Soderberg I,Lindholm M,et al. Inhibition of atherosclerosis in apoE-null mice by immunization with apoB-100 peptide sequences. Arterioscler Thromb Vasc Biol,2003,23(5):879-884.
109.Lichtman AH,Binder CJ,Tsimikas S,et al. Adaptive immunity in atherogenesis:new insights and therapeutic approaches. J Clin Invest,2013,123(1):27-36.
110.Fredrikson GN,Schiopu A,Berglund G,et al. Autoantibody against the amino acid sequence 661-680 in apo B-100 is associated with decreased carotid stenosis and cardiovascular events. Atherosclerosis,2007,194(2):e188-e192.
111.Fredrikson GN,Bjorkbacka H,Soderberg I,et al. Treatment with apo B peptide vaccines inhibits atherosclerosis in human apo B-100 transgenic mice without inducing an increase in peptide-specific antibodies. J Intern Med,2008,264(6):563-570.
112.Pierides C,Bermudez-Fajardo A,Fredrikson GN,et al. Immune responses elicited by apoB-100-derived peptides in mice. Immunol Res,2013,56(1):96-108.
113.Hermansson A,Johansson DK,Ketelhuth DF,et al. Immunotherapy with tolerogenic apolipoprotein B-100-loaded dendritic cells attenuates atherosclerosis in hypercholesterolemic mice. Circulation,2011,123(10):1083-1091.
114.Tse K,Gonen A,Sidney J,et al. Atheroprotective Vaccination with MHC-Ⅱ Restricted Peptides from ApoB-100. Front Immunol,2013,4:493.
115.Kimura T,Tse K,Sette A,et al. Vaccination to modulate atherosclerosis. Autoimmunity,2015,48(3):152-160.
116.Zhou L,Hussain MM. Human MicroRNA-548p Decreases Hepatic Apolipoprotein B Secretion and Lipid Synthesis. Arterioscler Thromb Vasc Biol,2017,37(5):786-793.
117.Hsu SH,Wang B,Kota J,et al. Essential metabolic,anti-inflammatory,and anti-tumorigenic functions of miR-122 in liver. J Clin Invest,2012,122(8):2871-2883.
118.Xu Y,Zalzala M,XuJ,et al. A metabolic stress-inducible miR-34a-HNF4alpha pathway regulates lipid and lipoprotein metabolism. Nat Commun,2015,6:7466.
119.Soh J,Iqbal J,Queiroz J,et al. MicroRNA-30c reduces hyperlipidemia and atherosclerosis in mice by decreasing lipid synthesis and lipoprotein secretion. Nat Med,2013,19(7):892-900.
120.Momtazi AA,Banach M,Pirro M,et al. MicroRNAs:New Therapeutic Targets for Familial Hypercholesterolemia ?. Clin Reviews Allergy Immunol,2017.